Contents
- 1
"Genome: An autobiography of a species in 23 chapters" by Mat Ridley
- 1.1 P R E F A C E
- 1.2 C H R O M O S O M E 1
- 1.3 C H R O M O S O M E 2
- 1.4 C H R O M O S O M E 3
- 1.5 C H R O M O S O M E 4
- 1.6 C H R O M O S O M E 6
- 1.7 C H R O M O S O M E 7
- 1.8 C H R O M O S O M E S X AND Y
- 1.9 C H R O M O S O M E 8
- 1.10 C H R O M O S O M E 9
- 1.11 C H R O M O S O M E 1 0
- 1.12 C H R O M O S O M E 1 1
- 1.13 C H R O M O S O M E 1 2
- 1.14 C H R O M O S O M E 13
- 1.15 C H R O M O S O M E 1 4
- 1.16 C H R O M O S O M E 1 5
- 1.17 C H R O M O S O M E 16
- 1.18 C H R O M O S O M E 1 7
- 1.19 C H R O M O S O M E 18
- 1.20 C H R O M O S O M E 1 9
- 1.21 CHROMOSOME 21
- 1.22 CHROMOSOME 22
"Genome: An autobiography of a species in 23 chapters" by Mat Ridley
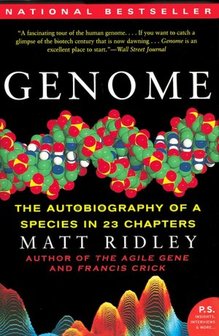
P R E F A C E
The human genome — the complete set of human genes - comes packaged in twenty-three separate pairs of chromosomes. Of these, twenty-two pairs are numbered in approximate order of size, from the largest (number 1) to the smallest (number 22), while the remaining pair consists of the sex chromosomes: two large X chromosomes in women, one X and one small Y in men. In size, the X comes between chromosomes 7 and 8, whereas the Y is the smallest. The number 23 is of no significance. Many species, including our closest relatives among the apes, have more chromosomes, and many have fewer. Nor do genes of similar function and type necessarily cluster on the same chromosome. Chromosomes are, after all, merely arbitrary collections of genes.
The human body contains approximately 100 trillion CELLS, most of which are less than a tenth of a millimetre across. Inside each cell there is a black blob called a NUCLEUS. Inside the nucleus are two complete sets of the human GENOME (except in egg cells and sperm cells, which have one copy each, and red blood cells, which have none). One set of the genome came from the mother and one from the father. In principle, each set includes the same 60,000-80,000 GENES on the same twenty-three CHROMOSOMES. In practice, there are often small and subtle differences between the paternal and maternal versions of each gene, differences that account for blue eyes or brown, for example. When we breed, we pass on one complete set, but only after swapping bits of the paternal and maternal chromosomes in a procedure known as RECOMBINATION.
Imagine that the genome is a book.
There are twenty-three chapters, called CHROMOSOMES.
Each chapter contains several thousand stories, called GENES.
Each story is made up of paragraphs, called EXONS, which are interrupted by advertisements called INTRONS.
Each paragraph is made up of words, called CODONS.
Each word is written in letters called BASES.
There are one billion words in the book, which makes it longer than 5,000 volumes the size of this one, or as long as 800 Bibles. If I read the genome out to you at the rate of one word per second for eight hours a day, it would take me a century. If I wrote out the human genome, one letter per millimetre, my text would be as long as the River Danube. This is a gigantic document, an immense book, a recipe of extravagant length, and it all fits inside the microscopic nucleus of a tiny cell that fits easily upon the head of a pin. The idea of the genome as a book is not, strictly speaking, even a metaphor. It is literally true. A book is a piece of digital information, written in linear, one-dimensional and one-directional form and defined by a code that transliterates a small alphabet of signs into a large lexicon of meanings through the order of their groupings. So is a genome. The only complication is that all English books read from left to right, whereas some parts of the genome read from left to right, and some from right to left, though never both at the same time.
C H R O M O S O M E 1
L i f e
Life is a slippery thing to define, but it consists of two very different skills: the ability to replicate, and the ability to create order. Living things produce approximate copies of themselves: rabbits produce rabbits, dandelions make dandelions. But rabbits do more than that. They eat grass, transform it into rabbit flesh and somehow build bodies of order and complexity from the random chaos of the world. They do not defy the second law of thermodynamics, which says that in a closed system everything tends from order towards disorder, because rabbits are not closed systems. Rabbits build packets of order and complexity called bodies but at the cost of expending large amounts of energy. In Erwin Schrodinger's phrase, living creatures 'drink orderliness' from the environment. The key to both of these features of life is information. The ability to replicate is made possible by the existence of a recipe, the information that is needed to create a new body. A rabbit's egg carries the instructions for assembling a new rabbit.
An adult rabbit, with its ability to both reproduce and metabolise, is prefigured and presupposed in its living filaments in the same way that a cake is prefigured and presupposed in its recipe. This is an idea that goes right back to Aristotle, who said that the 'concept' of a chicken is implicit in an egg, or that an acorn was literally 'informed' by the plan of an oak tree.
Go back not to 1953, the year of the discovery of DNA' s symmetrical structure, but ten years further, to 1943. Those who will do most to crack the mystery, a whole decade later, are working on other things in 1943. Francis Crick is working on the design of naval mines near Portsmouth. At the same time James Watson is just enrolling as an undergraduate at the precocious age of fifteen at the University of Chicago; he is determined to devote his life to ornithology. Maurice Wilkins is helping to design the atom bomb in the United States. Rosalind Franklin is studying the structure of coal for the British government. In Auschwitz in 1943, Josef Mengele is torturing twins to death in a grotesque parody of scientific inquiry. Mengele is trying to understand heredity, but his eugenics proves not to be the path to enlightenment. Mengele's results will be useless to future scientists. In Dublin in 1943, a refugee from Mengele and his ilk, the great physicist Erwin Schrodinger is embarking on a series of lectures at Trinity College entitled What is life?' He is trying to define a problem. He knows that chromosomes contain the secret of life, but he cannot understand how: 'It is these chromosomes . . . that contain in some kind of code-script the entire pattern of the individual's future development and of its functioning in the mature state.' The gene, he says, is too small to be anything other than a large molecule, an insight that will inspire a generation of scientists, including Crick, Watson, Wilkins and Franklin, to tackle what suddenly seems like a tractable problem. Having thus come tantalisingly close to the answer, though, Schrodinger veers off track. He thinks that the secret of this molecule's ability to carry heredity lies in his beloved quantum theory, and is pursuing that obsession down what will prove to be a blind alley. The secret of life has nothing to do with quantum states. The answer will not come from physics. In New York in 1943, a sixty-six-year-old Canadian scientist, Oswald Avery, is putting the finishing touches to an experiment that will decisively identify DNA as the chemical manifestation of heredity. He has proved in a series of ingenious experiments that a pneumonia bacterium can be transformed from a harmless to a virulent strain merely by absorbing a simple chemical solution. By 1943, Avery has concluded that the transforming substance, once purified, is DNA. But he will couch his conclusions in such cautious language for publication that few will take notice until much later. In a letter to his brother Roy written in May 1943, Avery is only slightly less cautious.
Avery is almost there, but he is still thinking along chemical lines. That life is chemistry is true but boring, like saying that football is physics. Life, to a rough approximation, consists of the chemistry of three atoms, hydrogen, carbon and oxygen, which between them make up ninety-eight per cent of all atoms in living beings. But it is the emergent properties of life — such as heritability - not the constituent parts that are interesting.
The answer will not come from chemistry. In Bletchley, in Britain, in 1943, in total secrecy, a brilliant mathematician,Alan Turing, is seeing his most incisive insight turned into physical reality. Turing has argued that numbers can compute numbers. To crack the Lorentz encoding machines of the German forces, a computer called Colossus has been built based on Turing's principles: it is a universal machine with a modifiable stored program. Nobody realises it at the time, least of all Turing, but he is probably closer to the mystery of life than anybody else.
Anything that can use the resources of the world to get copies of itself made is alive;
In New Jersey in 1943, a quiet, reclusive scholar named Claude Shannon is ruminating about an idea he had first had at Princeton a few years earlier. Shannon's idea is that information and entropy are opposite faces of the same coin and that both have an intimate link with energy. The less entropy a system has, the more information it contains. The reason a steam engine can harness the energy from burning coal and turn it into rotary motion is because the engine has high information content — information injected into it by its designer. So does a human body.
Life, too, is digital information written in DNA
Imagine the nucleus of a human egg beneath the microscope. Arrange the twenty-three chromosomes, if you can, in order of size, the biggest on the left and the smallest on the right. Now zoom in on the largest chromosome, the one called, for purely arbitrary reasons, chromosome 1. Every chromosome has a long arm and a short arm separated by a pinch point known as a centromere. On the long arm of chromosome 1, close to the centromere, you will find, if you read it carefully, that there is a sequence of 120 letters - As, Cs, Gs and Ts - that repeats over and over again. Between each repeat there lies a stretch of more random text, but the 120-letter paragraph keeps coming back like a familiar theme tune, in all more than 100 times. This short paragraph is perhaps as close as we can get to an echo of the original word. This 'paragraph' is a small gene, probably the single most active gene in the human body. Its 120 letters are constantly being copied into a short filament of RNA. The copy is known as 5S RNA. It sets up residence with a lump of proteins and other RNAs, carefully intertwined, in a ribosome, a machine whose job is to translate DNA recipes into proteins. And it is proteins that enable DNA to replicate.
Protein represents chemistry, living, breathing, metabolism and behaviour - what biologists call the phenotype. DNA represents information, replication, breeding, sex - what biologists call the genotype.
But above all, RNA — unlike DNA — can act as a catalyst, breaking up and joining other molecules including RNAs themselves. It can cut them up, join the ends together, make some of its own building blocks, and elongate a chain of RNA. It can even operate on itself, cutting out a chunk of text and splicing the free ends together again. The discovery of these remarkable properties of RNA in the early 1980s, made by Thomas Cech and Sidney Altman, transformed our understanding of the origin of life. It now seems probable that the very first gene, the 'ur-gene', was a combined replicator—catalyst, a word that consumed the chemicals around it to duplicate itself. It may well have been made of RNA. By repeatedly selecting random RNA molecules in the test tube based on their ability to catalyse reactions, it is possible to 'evolve' catalytic RNAs from scratch —almost to rerun the origin of life. And one of the most surprising results is that these synthetic RNAs often end up with a stretch of RNA text that reads remarkably like part of the text of a ribosomal RNA gene such as the 5S gene on chromosome 1. Back before the first dinosaurs, before the first fishes, before the first worms, before the first plants, before the first fungi, before the first bacteria, there was an RNA world — probably somewhere around four billion years ago, soon after the beginning of planet earth's very existence and when the universe itself was only ten billion years old. We do not know what these 'ribo-organisms' looked like. We can only guess at what they did for a living, chemically speaking. We do not know what came before them. We can be pretty sure that they once existed, because of the clues to RNA' s role that survive in living organisms today. These ribo-organisms had a big problem. RNA is an unstable substance, which falls apart within hours. Had these organisms ventured anywhere hot, or tried to grow too large, they would have faced what geneticists call an error catastrophe - a rapid decay of the message in their genes. One of them invented by trial and error a new and tougher version of RNA called DNA and a system for making RNA copies from it, including a machine we'll call the proto-ribosome. It had to work fast and it had to be accurate. So it stitched together genetic copies three letters at a time, the better to be fast and accurate. Each threesome came flagged with a tag to make it easier for the proto-ribosome to find, a tag that was made of amino acid. Much later, those tags themselves became joined together to make proteins and the three-letter word became a form of code for the proteins - the genetic code itself. (Hence to this day, the genetic code consists of three-letter words, each spelling out a particular one of twenty amino acids as part of a recipe for a protein.) And so was born a more sophisticated creature that stored its genetic recipe on DNA, made its working machines of protein and used RNA to bridge the gap between them. Her name was Luca, the Last Universal Common Ancestor.
There is, however, a conceptual difficulty about trying to identify the earliest forms of life. These days it is impossible for most creatures to acquire genes except from their parents, but that may not always have been so. Even today, bacteria can acquire genes from other bacteria merely by ingesting them. There might once have been widespread trade, even burglary, of genes. In the deep past chromosomes were probably numerous and short, containing just one gene each, which could be lost or gained quite easily. If this was so, Carl Woese points out, the organism was not yet an enduring entity. It was a temporary team of genes. The genes that ended up in all of us may therefore have come from lots of different 'species' of creature and it is futile to try to sort them into different lineages. We are descended not from one ancestral Luca, but from the whole community of genetic organisms. Life, says Woese, has a physical history, but not a genealogical one.
We are all descended from society, not from an individual species.
Those books assert that the first creatures were like bacteria, simple cells with single copies of circular chromosomes, and that all other living things came about when teams of bacteria ganged together to make complex cells. It may much more plausibly be the exact reverse.
The very first modern organisms were not like bacteria; they did not live in hot springs or deep-sea volcanic vents. They were much more like protozoa: with genomes fragmented into several linear chromosomes rather than one circular one, and 'polyploid' — that is, with several spare copies of every gene to help with the correction of spelling errors. Moreover, they would have liked cool climates. As Patrick Forterre has long argued, it now looks as if bacteria came later, highly specialised and simplified descendants of the Lucas, long after the invention of the DNA-protein world. Their trick was to drop much of the equipment of the RNA world specifically to enable them to live in hot places. It is we that have retained the primitive molecular features of the Lucas in our cells; bacteria are much more 'highly evolved' than we are. This strange tale is supported by the existence of molecular 'fossils'- little bits of RNA that hang about inside the nucleus of your cells doing unnecessary things such as splicing themselves out of genes: guide RNA, vault RNA, small nuclear RNA, small nucleolar RNA, self-splicing introns. Bacteria have none of these, and it is more parsimonious to believe that they dropped them rather than we invented them. (Science, perhaps surprisingly, is supposed to treat simple explanations as more probable than complex ones unless given reason to think otherwise; the principle is known in logic as Occam's razor.) Bacteria dropped the old RNAs when they invaded hot places like hot springs or subterranean rocks where temperatures can reach 170 °C — to minimise mistakes caused by heat, it paid to simplify the machinery.
We retained those old RNAs, relics of machines long superseded, but never entirely thrown away. Unlike the massively competitive world of bacteria, we — that is all animals, plants and fungi - never came under such fierce competition to be quick and simple. We put a premium instead on being complicated, in having as many genes as possible, rather than a streamlined machine for using them. The three-letter words of the genetic code are the same in every creature. CGA means arginine and GCG means alanine - in bats, in beetles, in beech trees, in bacteria. They even mean the same in the misleadingly named archaebacteria living at boiling temperatures in sulphurous springs thousands of feet beneath the surface of the Atlantic ocean or in those microscopic capsules of deviousness called viruses. Wherever you go in the world, whatever animal, plant, bug or blob you look at, if it is alive, it will use the same dictionary and know the same code. All life is one.
We have no fossil record of the way life was four billion years ago. We have only this great book of life, the genome. The genes in the cells of your little finger are the direct descendants of the first replicator molecules; through an unbroken chain of tens of billions of copyings, they come to us today still bearing a digital message that has traces of those earliest struggles of life.
C H R O M O S O M E 2
S p e c i e s
Natural selection is simply the process by which life-forms change to suit the myriad opportunities afforded by the physical environment and by other life-forms. The black-smoker bacterium, living in a sulphurous vent on the floor of the Atlantic ocean and descended from a stock of bacteria that parted company with our ancestors soon after Luca's day, is arguably more highly evolved than a bank clerk, at least at the genetic level. Given that it has a shorter generation time, it has had more time to perfect its genes.
Human beings are of course unique. They have, perched between their ears, the most complicated biological machine on the planet. But complexity is not everything, and it is not the goal of evolution. Every species on the planet is unique.
Human beings are an ecological success. They are probably the most abundant large animal on the whole planet.
Moreover, the human species has shown a remarkable capacity for colonising different habitats, cold or hot, dry or wet, high or low, marine or desert. Ospreys, barn owls and roseate terns are the only other large species to thrive in every continent except Antarctica and they remain strictly confined to certain habitats.
Yet the remarkable truth is that we come from a long line of failures. We are apes, a group that almost went extinct fifteen million years ago in competition with the better-designed monkeys. We are primates, a group of mammals that almost went extinct forty-five million years ago in competition with the better-designed rodents. We are synapsid tetrapods, a group of reptiles that almost went extinct 200 million years ago in competition with the better-designed dinosaurs. We are descended from limbed fishes, which almost went extinct 360 million years ago in competition with the better-designed ray-finned fishes. We are chordates, a phylum that survived the Cambrian era 500 million years ago by the skin of its teeth in competition with the brilliantly successful arthropods. Our ecological success came against humbling odds. In the four billion years since Luca, the word grew adept at building what Richard Dawkins has called 'survival machines': large, fleshy entities known as bodies that were good at locally reversing entropy the better to replicate the genes within them. They had done this by a venerable and massive process of trial and error, known as natural selection.
Three billion years during which trillions of trillions of single-celled creatures lived, each one reproducing and dying every few days or so, amounts to a big heap of trial and error. But it turned out that life was not finished. About a billion years ago, there came, quite suddenly, a new world order, with the invention of bigger, multicellular bodies, a sudden explosion of large creatures. Within the blink of a geological eye (the so-called Cambrian explosion may have lasted a mere ten or twenty million years), there were vast creatures of immense complexity: scuttling trilobites nearly a foot long; slimy worms even longer; waving algae half a yard across. Single-celled creatures still dominated, but these great unwieldy forms of giant survival machines were carving out a niche for themselves.
Apart from the fusion of chromosome 2, visible differences between chimp and human chromosomes are few and tiny. We are, to a ninety-eight per cent approximation, chimpanzees, and they are, with ninety-eight per cent confidence limits, human beings. If you took two Plasticene amoebae and turned one into a chimpanzee, the other into a human being, almost all the changes you would make would be the same. Both would need thirty-two teeth, five fingers, two eyes, four limbs and a liver. There is no bone in the chimpanzee body that I do not share.
In a last, desperate defence of his species against the theory of descent from the apes, the Victorian anatomist Sir Richard Owen once claimed that the hippocampus minor was a brain lobe unique to human brains, so it must be the seat of the soul and the proof of divine creation. He could not find the hippocampus minor in the freshly pickled brains of gorillas brought back from the Congo by the adventurer Paul du Chaillu. Thomas Henry Huxley furiously responded that the hippocampus minor was there in ape brains. 'No, it wasn't', said Owen. Was, too', said Huxley. Huxley, by the way, was right. After all, it is less than 300,000 human generations since the common ancestor of both species lived in central Africa.
Five million years is a long time, but evolution works not in years but in generations. Bacteria can pack in that many generations in just twenty-five years.
Whatever the mechanism, we can guess that our ancestors were a small, isolated band, while those of the chimpanzees were the main race. We can guess this because we know from the genes that human beings went through a much tighter genetic bottleneck (i.e., a small population size) than chimpanzees ever did: there is much less random variability in the human genome than the chimp genome.
C H R O M O S O M E 3
H i s t o r y
In his work at St Bartholomew's Hospital and Great Ormond Street in London, Garrod had come across a number of patients with a rare and not very serious disease, known as alkaptonuria. Among other more uncomfortable symptoms such as arthritis, their urine and the ear wax turned reddish or inky black on exposure to the air, depending on what they had been eating. In 1901, the parents of one of these patients, a little boy, had a fifth child who also had the affliction. That set Garrod to thinking about whether the problem ran in families. He noticed that the two children's parents were first cousins. So he went back and re-examined the other cases: three of the four families were first-cousin marriages, and of the seventeen alkaptonuria cases he saw, eight were second cousins of each other. But the affliction was not simply passed on from parent to child. Most sufferers had normal children, but the disease could reappear later in their descendants.
Garrod knew he was dealing with a Mendelian recessive - a character that could be carried by one generation but would only be expressed if inherited from both parents.
Thus was born Garrod's bold hypothesis of the 'inborn errors of metabolism', with its far-reaching assumption that genes were there to produce chemical catalysts, one gene to each highly specialised catalyst. Perhaps that was what genes were: devices for making proteins. 'Inborn errors of metabolism', Garrod wrote, 'are due to the failure of a step in the metabolic sequence due to loss or malfunction of an enzyme.' Since enzymes are made of protein, they must be the 'seat of chemical individuality'. Garrod's book, published in 1909, was widely and positively reviewed, but his reviewers comprehensively missed the point. They thought he was talking about rare diseases, not something fundamental to all life.
We now know that the main purpose of genes is to store the recipe for making proteins. It is proteins that do almost every chemical, structural and regulatory thing that is done in the body: they generate energy, fight infection, digest food, form hair, carry oxygen and so on and on. Every single protein in the body is made from a gene by a translation of the genetic code.
Garrod's conjecture is basically correct: what we inherit from our parents is a gigantic list of recipes for making proteins and for making protein-making machines - and little more.
Christened Johann Mendel, he was born in the tiny village of Heinzendorf (now Hynoice) in Northern Moravia in 1822. His father, Anton, was a smallholder who paid his rent in work for his landlord; his health and livelihood were shattered by a falling tree when Johann was sixteen and doing well at the grammar school in Troppau. Anton sold the farm to his son-in-law so he could afford the fees for his son at school and then at university in Olmiitz. But it was a struggle and Johann needed a wealthier sponsor, so he became an Augustinian friar, taking the name Brother Gregor. He trundled through theological college in Brunn (now Brno) and emerged a priest. He did a stint as a parish priest, but it was not a success. He tried to become a science teacher after studying at Vienna University, but failed the examination. Back to Brunn he went, a thirty-one-year-old nonentity, fit only for monastic life. He was good at mathematics and chess playing, had a decent head for figures and possessed a cheerful disposition. He was also a passionate gardener, having learnt from his father how to graft and breed fruit trees. It is here, in the folk knowledge of the peasant culture, that the roots of his insight truly lay. The rudiments of particulate inheritance were dimly understood already by the breeders of cattle and apples, but nobody was being systematic.
So Father Mendel, aged thirty-four, started a series of experiments on peas in the monastery gardens that were to last eight years, involve the planting of over 30,000 different plants - 6,000 in 1860 alone - and eventually change the world forever. In the garden, Mendel had been hybridising: crossing different varieties of pea plant. But this was no amateur gardener playing at science; this was a massive, systematic and carefully thought-out experiment. Mendel chose seven pairs of varieties of peas to cross. He crossed round-seeded peas with wrinkled ones; yellow cotyledons with green ones; inflated seed pods with wrinkled seed pods; grey seed coats with white seed coats; green unripe pods with yellow unripe pods; axial flowers with terminal flowers; tall stems with dwarf stems.
In every case, the resulting hybrids were always like just one parent. The other parent's essence seemed to have vanished. But it had not: Mendel allowed the hybrids to self-fertilise and the essence of the missing grandparent reappeared intact in roughly one-quarter of the cases. He counted and counted - 19,959 plants in the second generation, with the dominant characters outnumbering the recessives by 14,949 to 5,010, or 2.98 to 1.
Like a man possessed, Mendel turned from peas to fuschias, maize and other plants. He found the same results. He knew that he had discovered something profound about heredity: characteristics do not mix. There is something hard, indivisible, quantum and particulate at the heart of inheritance. There is no mingling of fluids, no blending of blood; there is instead a temporary joining together of lots of little marbles. In retrospect, this was obvious all along. How else could people account for the fact that a family might contain a child with blue eyes and a child with brown? Darwin, who none the less based his theory on blending inheritance, hinted at the problem several times. 'I have lately been inclined to speculate', he wrote to Huxley in 1857, 'very crudely and indistinctly, that propagation by true fertilisation will turn out to be a sort of mixture, and not true fusion, of two distinct individuals . . .
Darwin was not a little nervous on the subject. He had recently come under attack from a fierce Scottish professor of engineering, strangely named Fleeming Jenkin, who had pointed out the simple and unassailable fact that natural selection and blending inheritance did not mix. If heredity consisted of blended fluids, then Darwin's theory probably would not work, because each new and advantageous change would be lost in the general dilution of descent. Jenkin illustrated his point with the story of a white man attempting to convert an island of black people to whiteness merely by breeding with them. His white blood would soon be diluted to insignificance. In his heart Darwin knew Jenkin was right, and even the usually ferocious Thomas Henry Huxley was silenced by Jenkin's argument, but Darwin also knew that his own theory was right. He could not square the two. If only he had read Mendel.
For four years, starting in 1866, Mendel sent his papers and his ideas to Karl-Wilhelm Nageli, professor of botany in Munich. With increasing boldness he tried to point out the significance of what he had found. For four years Nageli missed the point. He wrote back to the persistent monk polite but patronising letters, and told him to try breeding hawkweed. He could not have given more mischievous advice if he tried: hawkweed is apomictic, that is it needs pollen to breed but does not incorporate the genes of the pollinating partner, so cross-breeding experiments give strange results. After struggling with hawkweed Mendel gave up and turned
to bees. The results of his extensive experiments on the breeding of bees have never been found. Did he discover their strange 'haplodiploid' genetics?
Muller's great discovery, for which he was to win the Nobel prize, was that genes are artificially mutable.
By bombarding fruit flies with X-rays, Muller caused their genes to mutate so that their offspring sported new deformities.
Like atoms, Mendel's particles must have some internal structure, too. They could be changed by X-rays. They were still genes after mutation, but not the same genes.
Using Muller's X-rays, in 1940 two scientists named George Beadle and Edward Tatum created mutant versions of a bread mould called Neurospora. They then worked out that the mutants failed to make a certain chemical because they lacked the working version of a certain enzyme. They proposed a law of biology, which caught on and has proved to be more or less correct: one gene specifies one enzyme.
Muller, meanwhile, was out of the picture. In 1932 his fervent socialism and his equally fervent belief in the selective breeding of human beings, eugenics (he wanted to see children carefully bred with the character of Marx or Lenin, though in later editions of his book he judiciously altered this to Lincoln and Descartes), led him across the Atlantic to Europe. He arrived in Berlin just a few months before Hitler came to power. He watched, horrified, as the Nazis smashed the laboratories of his boss, Oscar Vogt, for not expelling the Jews under his charge. Muller went east once more, to Leningrad, arriving in the laboratory of Nikolay Vavilov just before the anti-Mendelist Trofim Lysenko caught the ear of Stalin and began his persecution of Mendelian geneticists in support of his own crackpot theories that wheat plants, like Russian souls, could be trained rather than bred to new regimes; and that those who believed otherwise should not be persuaded, but shot. Vavilov died in prison. Ever hopeful, Muller sent Stalin a copy of his latest eugenic book, but hearing it had not gone down well, found an excuse to get out of the country just in time. He went to the Spanish Civil War, where he worked in the blood bank of the International Brigade, and thence to Edinburgh,
arriving with his usual ill luck just in time for the outbreak of the Second World War. He found it hard to do science in a blacked-out Scottish winter wearing gloves in the laboratory and he tried desperately to return to America. But nobody wanted a belligerent, prickly socialist who lectured ineptly and had been living in Soviet Russia. Eventually Indiana University gave him a job. The following year he won the Nobel prize for his discovery of artificial mutation.
True, there was something else in chromosomes: that dull little nucleic acid called DNA. It had first been isolated, from the pus-soaked bandages of wounded soldiers, in the German town of Tubingen in 1869 by a Swiss doctor named Friedrich Miescher.
Drawn by the presence of Muller, there arrived in Bloomington, Indiana, a precocious and confident nineteen-year-old, already equipped with a bachelor's degree, named James Watson. He must have seemed an unlikely solution to the gene problem, but the solution he was. Trained at Indiana University by the Italian emigre Salvador Luria (predictably, Watson did not hit it off with Muller), Watson developed an obsessive conviction that genes were made of DNA, not protein. In search of vindication, he went to Denmark, then, dissatisfied with the colleagues he found there, to Cambridge in October 1951. Chance threw him together in the Cavendish laboratory with a mind of equal brilliance captivated by the same conviction about the importance of DNA, Francis Crick.
But he did not have the patience to stick to his own problems, or the humility to stick to small questions. His laugh, his confident intelligence and his knack of telling people the answers to their own scientific questions were getting on nerves at the Cavendish.
So was born one of the great, amicably competitive and therefore productive collaborations in the history of science: the young, ambitious, suppleminded American who knew some biology and the effortlessly brilliant but unfocused older Briton who knew some physics. It was an exothermic reaction. Within a few short months, using other people's laboriously gathered but under-analysed facts, they had made possibly the greatest scientific discovery of all time, the structure of DNA. Not even Archimedes leaping from his bath had been granted greater reason to boast, as Francis Crick did in the Eagle pub on 28 February 1953, 'We've discovered the secret of life.' Watson was mortified; he still feared that they might have made a mistake. But they had not. All was suddenly clear: DNA contained a code written along the length of an elegant, intertwined staircase of a double helix, of potentially infinite length. That code copied itself by means of chemical affinities between its letters and spelt out the recipes for proteins by means of an as yet unknown phrasebook linking DNA to protein.
A month after the Watson-Crick structure was published, Britain crowned a new queen and a British expedition conquered Mount Everest on the same day. Apart from a small piece in the News Chronicle, the double helix did not make the newspapers.
Finding the code had been, for Watson and Crick, almost easy — a mixture of guesswork, good physics and inspiration. Cracking the code required true brilliance.
Most of the best ideas that led to the answer came from Crick, including what he called the adaptor molecule - what we now call transfer RNA. Independendy of all evidence, Crick arrived at the conclusion that such a molecule must exist. It duly turned up. But Crick also had an idea that was so good it has been called the greatest wrong theory in history. Crick's 'comma-free' code is more elegant than the one Mother Nature uses. It works like this. Suppose that the code uses three letters in each word (if it uses two, that only gives sixteen combinations, which is too few). Suppose that it has no commas, and nogapsbetweenthewords. Now suppose that it excludes all words that can be misread if you start in the wrong place. So, to take an analogy used by Brian Hayes, imagine all three-letter English words that can be written with the four letters A, S, E and T: ass, ate, eat, sat, sea, see, set, tat, tea and tee. Now eliminate those that can be misread as another word if you start in the wrong place. For example, the phrase ateateat can be misread as 'a tea tea t' or as 'at eat eat' or as 'ate ate at'. Only one of these three words can survive in the code. Crick did the same with A, C, G and T. He eliminated AAA, CCC, GGG and TTT for a start. He then grouped the remaining sixty words into threes, each group containing the same three letters in the same rotating order. For example, ACT, CTA and TAC are in one group, because C follows A, T follows C, and A follows T in each; while ATC, TCA and CAT are in another group. Only one word in each group survived. Exactly twenty are left - and there are twenty amino acid letters in the protein alphabet! A fourletter code gives a twenty-letter alphabet.
But the time for theorising was past. In 1961, while everybody else was thinking, Marshall Nirenberg and Johann Matthaei decoded a 'word' of the code by the simple means of making a piece of RNA out of pure U (uracil - the equivalent of DNA' s T) and putting it in a solution of amino acids. The ribosomes made a protein by stitching together lots of phenylalanines. The first word of the code had been cracked: UUU means phenylalanine. The commafree code was wrong, after all. Its great beauty had been that it cannot have what are called reading-shift mutations, in which the loss of one letter makes nonsense of all that follows. Yet the version that Nature has instead chosen, though less elegant, is more tolerant of other kinds of errors. It contains much redundancy with many different three-letter words meaning the same thing.
C H R O M O S O M E 4
F a t e
The gene contains a single 'word', repeated over and over again: CAG, CAG, CAG, CAG . . . The repetition continues sometimes just six times, sometimes thirty, sometimes more than a hundred times. Your destiny, your sanity and your life hang by the thread of this repetition. If the 'word' is repeated thirty-five times or fewer, you will be fine.
The age at which the madness will appear depends strictly and implacably on the number of repetitions of the 'word' CAG in one place in one gene.
The scale is this: if your chromosomes were long enough to stretch around the equator, the difference between health and insanity would be less than one extra inch. No horoscope matches this accuracy. No theory of human causality, Freudian, Marxist, Christian or animist, has ever been so precise. No prophet in the Old Testament, no entrail-gazing oracle in ancient Greece, no crystal-ball gipsy clairvoyant on the pier at Bognor Regis ever pretended to tell people exactly when their lives would fall apart, let alone got it right. We are dealing here with a prophecy of terrifying, cruel and inflexible truth. There are a billion three-letter 'words' in your genome. Yet the length of just this one little motif is all that stands between each of us and mental illness. Huntington's disease, which became notorious when it killed the folk singer Woody Guthrie in 1967, was first diagnosed by a doctor, George Huntington, in 1872 on the eastern tip of Long Island. He noticed that it seemed to run in families. Later work revealed that the Long Island cases were part of a much larger family tree originating
in New England. In twelve generations of this pedigree more than a thousand cases of the disease could be found. All were descended from two brothers who emigrated from Suffolk in 1630. Several of their descendants were burnt as witches in Salem in 1693, possibly because of the alarming nature of the disease. But because the mutation only makes itself manifest in middle age, when people have already had children, there is little selective pressure on it to
die out naturally. Indeed, in several studies, those with the mutations appear to breed more prolifically than their unaffected siblings. Huntington's was the first completely dominant human genetic disease to come to light. That means it is not like alkaptonuria in which you must have two copies of the mutant gene, one from each parent, to suffer the symptoms. Just one copy of the mutation will do.
In the late 1970s, a determined woman set out to find the Huntington gene. Acting on the report of a Venezuelan doctor, Americo Negrette, in 1979 she flew to Venezuela to visit three rural villages called San Luis, Barranquitas and Laguneta on the shores of Lake Maracaibo. The area contained a vast, extended family with a high incidence of Huntington's disease. The story they told each other was that the affliction came from an eighteenth-century sailor, and Wexler was able to trace the family tree of the disease back to the early nineteenth century and a woman called, appropriately, Maria Conception. She lived in the Pueblos de Agua, villages of houses built on stilts over the water. A fecund ancestor, she had 11,000 descendants in eight generations, 9,000 of whom were still alive in 1981. No less than 371 of them had Huntington's disease when Wexler first visited and 3,600 carried a risk of at least a quarter that they would develop the disease, because at least one grandparent had the symptoms. Wexler's courage was extraordinary, given that she too might have the mutation. 'It is crushing to look at these exuberant children', she wrote,4 'full of hope and expectation, despite poverty, despite illiteracy, despite dangerous and exhausting work for the boys fishing in small boats in the turbulent lake, or for even the tiny girls tending house and caring for ill parents, despite a brutalising disease robbing them of parents, grandparents, aunts, uncles, and cousins - they are joyous and wild with life, until the disease attacks.'
The most unexpected feature of the stuttering repetition of the word CAG is that it is not confined to Huntington's disease. There are five other neurological diseases caused by so-called 'unstable CAG repeats' in entirely different genes
Moreover, there are other diseases of nerve degeneration caused by other stuttering repeats of 'words' and in every case the repeated 'word' begins with C and ends in G.
C H R O M O S O M E 6
I n t e l l i g e n c e
There is also some genuinely objective evidence for 'g': the speed with which people perform tasks involving the scanning and retrieval of information correlates with their IQ. And general IQ remains surprisingly constant at different ages: between six and eighteen, your intelligence increases rapidly, of course, but your IQ relative to your peers changes very little.
The same person tested twice 87
Identical twins reared together 86
Identical twins reared apart 76
Fraternal twins reared together 5 5
Biological siblings 47
Parents and children living together 40
Parents and children living apart 31
Adopted children living together 0
Unrelated people living apart 0
James Flynn, noticed in the 1980s that IQ is increasing in all countries all the time, at an average rate of about three IQ points per decade.
One scientist, Ulric Neisser, believes that the cause of the Flynn effect is the intense modern saturation of everyday life with sophisticated visual images — cartoons, advertisements, films, posters, graphics and other optical displays — often at the expense of written messages. Children experience a much richer visual environment than once they did, which helps develop their skills in visual puzzles of the kind that dominate IQ tests.
As Flynn himself notes, an increase of fifteen IQ points in five decades implies either that the world was full of dunces in 1950 or that it is full of geniuses today. Since we are not experiencing a cultural renaissance, he concludes that IQ measures nothing innate. But if Neisser is right, then the modern world is an environment that encourages the development of one form of intelligence - facility with visual symbols.
C H R O M O S O M E 7
I n s t i n c t
In one case, in Nicaragua, special schools for the deaf, established for the first time in the 1980s, led to the invention, de novo, of a whole new language. The schools taught lip-reading with little success, but in the playground the children brought together the various hand signs they used at home and established a crude pidgin language. Within a few years, as younger children learnt this pidgin, it was transformed into a true sign language with all the complexity, economy, efficiency and grammar of a spoken language. Once again, it was children who made the language, a fact that seems to suggest that the language instinct is one that is switched off as the child reaches adulthood.
For example, if Gopnik shows somebody a cartoon of an imaginary creature and with it the words 'This is a Wug', then shows them a picture of two such creatures together with the words 'These are . . .', most people reply, quick as a flash, 'Wugs'. Those with SLI rarely do so, and if they do, it is after careful thought. The English plural rule, that you add an 's' to the end of most words, is one they seem not to know. This does not prevent those with SLI knowing the plural of most words, but they are stumped by novel words that they have not seen before, and they make the mistake of adding 's' to fictitious words that the rest of us would not, such as 'saess'. Gopnik hypothesises that they store English plurals in their minds as separate lexical entries, in the same way that we all store singulars. They do not store the grammatical rule.
C H R O M O S O M E S X AND Y
C o n f l i c t
Far-fetched? Not at all. In the butterfly Acrea encedon, that is exactly what has happened. The sex ratio is ninety-seven per cent female as a result. This is just one of many cases known of this form of evolutionary conflict, known as sex-chromosome drive. Most known instances are confined to insects, but only because scientists have looked more closely at insects.
So effectively has the human Y chromosome shut down most of its genes that the great bulk of its length consists of non-coding DNA, serving no purpose at all - but giving few targets for the X chromosome genes to aim at.
C H R O M O S O M E 8
S e l f - i n t e r e s t
Actually, with the advent of email, the analogy is no longer as farfetched as it once was. Suppose I sent you an email that read: 'Beware, there is a nasty computer virus about; if you open a message with the word "marmalade" in the title, it will erase your hard disk! Please pass this warning on to everybody you can think of.' I made up the bit about the virus; there are, so far as I know, no emails called 'marmalade' doing the rounds. But I have very effectively hijacked your morning and caused you to send on my warning. My email was the virus.
Genes, remember, are stretches of DNA that comprise the recipe for proteins. But ninety-seven per cent of our genome does not consist of true genes at all. It consists of a menagerie of strange entities called pseudogenes, retropseudogenes, satellites, minisatellites, microsatellites, transposons and retrotransposons: all collectively known as 'junk DNA' , or sometimes, probably more accurately, as 'selfish DNA' . Some of these are genes of a special kind, but most are just chunks of DNA that are never transcribed into the language of protein.
Yet, ironically, junk DNA is the first part of the human genome that has found a real, practical, everyday use in the human world. It has led to DNA fingerprinting. Genes are protein recipes. But not all protein recipes are desirable. The commonest protein recipe in the entire human genome is the gene for a protein called reverse transcriptase. Reverse transcriptase is a gene that serves no purpose at all as far as the human body is concerned. If every copy of it were carefully and magically removed from the genome of a person at the moment of conception, the person's health, longevity and happiness would be more likely to be improved than damaged. Reverse transcriptase is vital for a certain kind of parasite. It is an extremely useful — nay essential - part of the genome of the AIDS virus: a crucial contributor to its ability to infect and kill its victims. For human beings, in contrast, the gene is a nuisance and a threat. Yet it is one of the commonest genes in the whole genome. There are several hundred copies of it, possibly thousands, spread about the human chromosomes. This is an astonishing fact, akin to discovering that the commonest use of cars is for getting away from crimes. Why is it there? A clue comes from what reverse transcriptase does. It takes an RNA copy of a gene, copies it back into DNA and stitches it back into the genome. It is a return ticket for a copy of a gene. By this means the AIDS virus can integrate a copy of its own genome into human DNA the better to conceal it, maintain it and get it efficiently copied. A good many of the copies of the reverse transcriptase gene in the human genome are there because recognisable 'retroviruses' put them there, long ago or even relatively recently. There are several thousand nearly complete viral genomes integrated into the human genome, most of them now inert or missing a crucial gene. These 'human endogenous retroviruses' or Hervs, account for 1.3% of the entire genome. That may not sound like much, but 'proper' genes account for only 3%.
A true genetic parasite. Such 'retrotransposons' are far commoner even than retroviruses. The commonest of all is a sequence of 'letters' known as a LINE-1. This is a 'paragraph' of DNA, between a thousand and six thousand 'letters' long, that includes a complete recipe for reverse transcriptase near the middle. LINE-1s are not only very common - there may be 100,000 copies of them in each copy of your genome — but they are also gregarious, so that the paragraph may be repeated several times in succession on the chromosome. They account for a staggering 14.6% of the entire genome, that is, they are nearly five times as common as 'proper' genes. The implications of this are terrifying. LINE-1s have their own return tickets. A single LINE-1 can get itself transcribed, make its own reverse transcriptase, use that reverse transcriptase to make a DNA copy of itself and insert that copy anywhere among the genes. This is presumably how there come to be so many copies of LINE- 1 in the first place. In other words, this repetitive 'paragraph' of 'text' is there because it is good at getting itself duplicated - no other reason.
Even commoner than LINE-1s are shorter 'paragraphs' called Alus. Each Alu contains between 180 and 280 'letters', and seems to be especially good at using other people's reverse transcriptase to get itself duplicated. The Alu text may be repeated a million times in the human genome - amounting to perhaps ten per cent of the entire 'book'.
We are full of digital chain letters and warnings about marmalade. Approximately thirtyfive per cent of human DNA consists of various forms of selfish DNA, which means that replicating our genes takes thirty-five per cent more energy than it need.
There are worms in animals' guts, bacteria in their blood, viruses in their cells. Why not retrotransposons in their genes? Moreover, by the mid-1970s, it was dawning on many evolutionary biologists, especially those interested in behaviour, that evolution by natural selection was not much about competition between species, not much about competition between groups, not even mostly about competition between individuals, but was about competition between genes using individuals and occasionally societies as their temporary vehicles. For instance, given the choice between a safe, comfortable and long life for the individual or a risky, tiring and dangerous attempt to breed, virtually all animals (and indeed plants) choose the latter. They choose to shorten their odds of death in order to have offspring. Indeed, their bodies are designed with planned obsolescence called ageing that causes them to decay after they reach breeding age — or, in the case of squid or Pacific salmon, to die at once. None of this makes any sense unless you view the body as a vehicle for the genes, as a tool used by genes in their competition to perpetuate themselves. The body's survival is secondary to the goal of getting another generation started.
To explain the fact that the genome contained huge gene-less regions, two pairs of scientists suggested in 1980 that these regions were replete with selfish sequences whose only function was survival within the genome.
Genes do indeed behave as if they have selfish goals, not consciously, but retrospectively: genes that behave in this way thrive and genes that don't don't.
In human beings, LINE-1s and Alus have caused mutations by landing in the middle of all sorts of genes. They have caused haemophilia, for instance, by landing in clotting-factor genes. But, for reasons that are not well understood, as a species we are less troubled by DNA parasites than some other species. Approximately 1 in every 700 human mutations is caused by 'jumping genes', whereas in mice nearly ten per cent of mutations are caused by jumping genes. The potential danger posed by jumping genes was dramatically illustrated by a sort of natural experiment in the 1950s in the tiny fruit fly, Drosophila. The fruit fly is the favourite experimental animal for geneticists. The species they study, called Drosophila melanogaster, has been transported all over the world to be bred in laboratories. It has frequently escaped and has met other, native species of fruit fly. One of these species, called Drosophila willistoni, carries a jumping gene called a P element. Somehow in about 1950, somewhere in South America, perhaps via a blood-sucking mite, Drosophila willistoni's jumping gene entered the Drosophila melanogaster species. (One of the great concerns attached to so-called 'xeno-transplants' of organs from pigs or baboons is that they might unleash a new form of jumping gene upon our species, like the P element of fruit flies.) The P element has since spread like wildfire, so that most fruit flies have the P element, though not those collected from the wild before 1950 and kept in isolation since. The P element is a piece of selfish DNA that shows its presence by disrupting the genes into which it jumps. Gradually, the rest of the genes in the fruit fly's genome have fought back, inventing ways of suppressing the P element's jumping habit. The P elements are settling down as passengers.
Human beings possess nothing so sinister as a P element, at least not at the moment.
What we see in the human genome is not some rapidly advancing parasitic infection, but the dormant cysts of many past parasites, each of which spread rapidly until the genome found a way of suppressing them, but not excising them. In this respect (as in others) we seem to be more fortunate than fruit flies. We appear to have a general mechanism for suppressing selfish DNA, at least if you believe a controversial new theory. The suppression mechanism goes by the name of cytosine methylation.
The new theory holds that during the early development of the embryo, all genes are briefly stripped of any methylation and switched on. This is then followed by a close inspection of the whole genome by molecules whose job is to spot repetitive sequences and close them down with methylation. In cancer tumours, one of the first things to happen is demethylation of the genes. As a result, the selfish DNA is released from its handcuffs and richly expressed in tumours.
LINE-1 is generally about 1,400 'letters' long. Alu is generally at least 180 'letters' long. There are, however, sequences even shorter than Alu that also accumulate in vast, repetitive stutters.
It is one of these short sequences that has a practical use in forensic and other sciences. Meet the 'hypervariable minisatellite'. This neat little sequence is found on all the chromosomes; it crops up at more than one thousand locations in the genome. In every case the sequence consists of a single 'phrase', usually about twenty 'letters' long, repeated over and over again many times.
Following instructions, the cell starts swapping the phrases with the equivalent series on the other copy of the same chromosome. But in doing so it makes fairly frequent mistakes, adding or subtracting to the number of repeats. In this way each series of repeats gradually changes length, fast enough so that it is different in every individual, but slowly enough so that people mostly have the same repeat lengths as their parents. Since there are thousands of series, the result is a unique set of numbers for each individual.
C H R O M O S O M E 9
D i s e a s e
People with type A blood could safely donate to those with A or AB; those with B could donate to those with B and AB; those with AB could donate only to those with AB; and those with O blood could donate to anybody - O is therefore known as the universal donor. Nor was there any obvious geographic or racial reason underlying the different types. Roughly forty per cent of Europeans have type O blood, forty per cent have type A blood, fifteen per cent have type B blood and five per cent have type AB blood. The proportions are similar in other continents, with the marked exception of the Americas, where the native American population was almost exclusively type O, save for some Canadian tribes, who were very often type A, and Eskimos, who were sometimes type AB or B.
The difference between the A gene and the B gene is seven letters out of 1,062, of which three are synonymous or silent: that is, they make no difference to the amino acid chosen in the protein chain. The four that matter are letters 523, 700, 793 and 800. In people with type A blood these letters read C, G, C, G. In people with type B blood they read G, A, A, C.
The O group has just a single spelling change compared with A, but instead of a substitution of one letter for another, it is a deletion. In people with type O blood, the 258th letter, which should read 'G', is missing altogether. The effect of this is far-reaching, because it causes what is known as a reading-shift or frame-shift mutation, which is far more consequential. (Recall that if Francis Crick's ingenious comma-free code of 1957 had been correct, reading-shift mutations would not have existed.) The genetic code is read in three-letter words and has no punctuation. An English sentence written in three-letter words might read something like: the fat cat sat top mat and big dog ran bit cat. Not exactly poetry, I admit, but it will do. Change one letter and it still makes fairly good sense: the fat xat sat top mat and big dog ran bit cat. But delete the same letter instead, and read the remaining letters in groups of three, and you render the whole sentence meaningless: the fat ats att opm ata ndb igd ogr anb itc at. This is what has happened to the ABO gene in people with the O blood group. Because they lack just one letter fairly early in the message, the whole subsequent message says something completely different. A different protein is made with different properties. The chemical reaction is not catalysed.
Indeed, the remarkable thing about blood groups, the thing that has made them so useful and so politically neutral, is that they seem to be completely invisible; they correlate with nothing.
At first glance the blood groups seem to be an example of the neutral theory of evolution, promulgated by Motoo Kimura in 1968: the notion that most genetic diversity is there because it makes no difference, not because it has been picked by natural selection for a purpose.
Remember that we each have two copies of each chromosome, so A people are actually AAs, that is they have an A gene on each of their ninth chromosomes, and B people are actually BBs. Now imagine a population with just these three kinds of blood groups: AA, BB and AB. The A gene is better for cholera resistance than the B gene. AA people are therefore likely to have more surviving children than BB people. Therefore the B gene is likely to die out - that's natural selection. But it doesn't happen like that, because AB people survive best of all. So the healthiest children will be the offspring of AAs and BBs. All their children will be AB, the most cholera-resistant type. But even if an AB mates with another AB, only half their children will be AB; the rest will be AA and BB, the latter being the most susceptible type. It is a world of strangely fluctuating fortunes.
The sickle-cell mutation, which causes blood cells to collapse in the absence of oxygen, is frequently fatal to those with two copies of it, but only mildly harmful to those with just one copy. But those with one copy are largely resistant to malaria.
Sickle-cell disease is a high price paid today for malaria resistance in the past.
Approximately one in five people are genetically unable to release the water-soluble form of the ABO blood group proteins into their saliva and other body fluids. These 'non-secretors' are more likely to suffer from various forms of disease, including meningitis, yeast infection and recurrent urinary tract infection. But they are less likely to suffer from influenza or respiratory syncitial virus. Wherever you look, the reasons behind genetic variability seem to have something to do with infectious disease. We have barely scratched the surface of this subject. As they scourged our ancestors, the great epidemic diseases of the past - plague, measles, smallpox, typhus, influenza, syphilis, typhoid, chicken pox, and others - left behind their imprint on our genes. Mutations which granted resistance thrived, but that resistance often came at a price, the price varying from severe (sickle-cell anaemia) to theoretical (the inability to receive transfusions of the wrong type of blood).
C H R O M O S O M E 1 0
S t r e s s
Cortisol is used in virtually every system in the body, a hormone that literally integrates the body and the mind by altering the configuration of the brain. Cortisol interferes with the immune system, changes the sensitivity of the ears, nose and eyes, and alters various bodily functions. When you have a lot of Cortisol coursing through your veins, you are - by definition — under stress.
Short-term stressors cause an immediate increase in epinephrine and norepinephrine, the hormones that make the heart beat faster, the feet go cold.
Stressors that last for longer activate a different pathway that results in a much slower, but more persistent increase in Cortisol. One of Cortisol's most surprising effects is that it suppresses the working of the immune system.
Those who lived near Three Mile Island nuclear plant at the time of its accident had more cancers than expected three years later, not because they were exposed to radiation (they weren't), but because their Cortisol levels had risen, reducing the responsiveness of their immune system to cancer cells.
People with most psychological stress in their past get more colds than people who have led happy lives.
You can trigger activity in the 'happiness centres' of the brain with a deliberate smile, as surely as you trigger a smile with happy thoughts. It really does make you feel better to smile.
By consistently choosing which males to mate with over many generations, female animals can alter the shape, colour, size or song of males of their species. Indeed, as I described in the chapter on chromosomes X and Y, Darwin suggested that this is exactly what has happened in the case of peacocks. It was not until a century later, in the 1970s and 1980s, that a series of theoretical and experimental studies demonstrated that Darwin was right, and that the tails, plumes, antlers, songs and size of male animals are bred into them by consistent trends of passive or active female choice, generation after generation.
C H R O M O S O M E 1 1
P e r s o n a l i t y
The gene for blue eyes is common in people who are bad at using chopsticks, but nobody would dream of suggesting that chopstick skill is genetically determined by the gene for eye colour. It just happens that both blue eyes and chopstick incompetence correlate with non-oriental origin for a blindingly obvious non-genetic reason called culture.
People with 'long' D4DR genes have low responsiveness to dopamine, so they need to take a more adventurous approach to life to get the same dopamine 'buzz' that short-gened people get from simple things.
Marriage counsellors, too, report good results from encouraging their clients to accept that they cannot change their partners' irritating habits - because they are probably innate - but must find ways to live with them.
Shy adolescents were more likely to be blue-eyed (all the subjects were of European descent), susceptible to allergies, tall and thin, narrow-faced, to have more heat-generating activity under the right forehead and a faster heartbeat, than the less shy individuals. All of these features are under the control of a particular set of cells in the embryo called the neural crest, from which a particular part of the brain, the amygdala, derives. They also all use the same neurotransmitter, called norepinephrine, a substance very like dopamine. All these features are also characteristic of northern Europeans, Nordic types for the most part. Kagan's argument goes that the Ice Age selected those better able to withstand cold in these parts: people with high metabolic rates. But a high metabolic rate is produced by an active norepinephrine system in the amygdala, and brings with it lots of different baggage - a phlegmatic and shy personality being one aspect and a pale appearance being another.
If you have unusually high levels of serotonin in your brain you will probably be a compulsive person, given to tidiness and caution, even to the point of being neurotic about it. People with the pathological condition known as obsessive—compulsive disorder can usually alleviate their symptoms by lowering their serotonin levels. At the other end of the spectrum, people with unusually low serotonin levels in their brains tend to be impulsive.
Increased serotonin alleviates anxiety and depression and can turn even fairly ordinary people into optimists
Indeed, even drugs and diets designed to lower blood cholesterol can influence serotonin. It is a curious fact that nearly all studies of cholesterol-lowering drugs and diets in ordinary people show an increase in violent death compared with control samples that usually matches the decrease in deaths from heart disease. In all studies put together, cholesterol treatment cut heart attacks by fourteen per cent, but raised violent deaths by an even more significant seventy-eight per cent. Because violent deaths are rarer than heart attacks, the numerical effect roughly cancels out, but violent deaths can sometimes involve innocent bystanders. So treating high cholesterol levels has its dangers. It has been known for twenty years that impulsive, antisocial and depressed people - including prisoners, violent offenders and failed suicides - have generally lower cholesterol levels than the population at large.
In the so-called MrFit trial, in which 351,000 people from seven countries were followed for seven years, people with very low cholesterol and people with very high cholesterol proved twice as likely to die at a given age as people with medium cholesterol. The extra deaths among low-cholesterol people are mainly due to accident, suicide or murder.
The link between low cholesterol and violence almost certainly involves serotonin. Monkeys fed on low-cholesterol diets become more aggressive and bad-tempered (even if they are not losing weight), and the cause seems to be a drop in serotonin levels.
Low serotonin is an accurate predictor of aggressiveness in monkeys, just as it is an accurate predictor of impulsive murder, suicide, fighting or arson in human beings.
Serotonin levels are not innate and inflexible. They are themselves the product of social status. The higher your selfesteem and social rank relative to those around you, the higher your serotonin level is. Experiments with monkeys reveals that it is the social behaviour that comes first. Serotonin is richly present in dominant monkeys and much more dilute in the brains of subordinates. Cause or effect? Almost everybody assumed the chemical was at least partly the cause: it just stands to reason that the dominant behaviour results from the chemical, not vice versa. It turns out to be the reverse: serotonin levels respond to the monkey's perception of its own position in the hierarchy, not vice versa. Contrary to what most people think, high rank means low aggressiveness, even in vervet monkeys. The high-ranking individuals are not especially large, fierce or violent. They are good at things like reconciliation and recruiting allies. They are notable for their calm demeanour. They are less impulsive, less likely to misinterpret playfighting as aggression.
There is little doubt that the monkey's mood is set by its high serotonin levels. If you artificially reverse the pecking order so that the monkey is now a subordinate, not only does its serotonin drop, but its behaviour changes, too. Moreover, much the same seems to happen in human beings. In university fraternities, the leading figures are blessed with rich serotonin concentrations which fall if they are deposed. Telling people they have low or high serotonin levels could become a self-fulfilling prophecy.
There is a Dutch family in which the men have been criminals for three generations, and the cause is undoubtedly a gene. The criminal men have an unusual version of a gene on the X chromosome called the monoamine oxidase A gene. Monoamine oxidase is responsible for breaking down serotonin among other chemicals.
C H R O M O S O M E 1 2
S e l f - A s s e m b l y
As the fertilised egg grows into an embryo, at first it is an undifferS entiated blob. Then gradually it develops two asymmetries - a headtail axis and a front-back axis. In fruit flies and toads, these axes are established by the mother, whose cells instruct one end of the embryo to become the head and one part to become the back. But in mice and people the asymmetries develop later and nobody knows quite how. The moment of implantation into the womb seems to be critical. In fruit flies and toads, these asymmetries are well understood: they consist of gradients in the chemical products of different maternal genes. In mammals, too, the asymmetries are almost certainly chemical. Each cell can, as it were, taste the soup inside itself, feed the information into its hand-held GPS microcomputer and get out a reading: 'you are in the rear half of the body, close to the underside.' Very nice to know where you are.
But knowing where you are is just the beginning. Knowing what you have to do once you are there is a wholly different problem. Genes that control this process are known as 'homeotic' genes. For instance, our cell, on discovering where it is located, looks this location up in its guidebook and finds the instruction: 'grow a wing', or 'start to become a kidney cell' or something like that. It is not of course literally like this. There are no computers and no guidebooks, just a series of automatic steps in which gene switches on gene which switches on gene. But a guidebook is a handy analogy, none the less, because the great beauty of embryo development, the bit that human beings find so hard to grasp, is that it is a totally decentralised process. Since every cell in the body carries a complete copy of the genome, no cell need wait for instructions from authority; every cell can act on its own information and the signals it receives from its neighbours.
There were 'gap' genes that had big effects, defining whole areas of the body, 'pair-rule' genes that subdivided these areas and defined finer details, and 'segment-polarity' genes that subdivided those details by affecting just the front or rear of a small section. The developmental genes seemed, in other words, to act hierarchically, parcelling up the embryo into smaller and smaller sections to create ever more detail.
This came as a great surprise. Until then, it had been assumed that the parts of the body defined themselves according to their neighbouring parts, not according to some grand genetic plan. But when the fruit-fly genes that had been mutated were pinned down and their sequences read, a further surprise was in store. The result was the first of two almost incredible discoveries, which between them amount to one of the most wonderful additions to knowledge of the twentieth century. The scientists found a cluster of eight homeotic genes lying together on the same chromosome, genes which became known as Hox genes. Nothing strange about that.
What was truly strange was that each of the eight genes affected a different part of the fly and they were lined up in the same order as the part of the fly they affected. The first gene affected the mouth, the second the face, the third the top of the head, the fourth the neck, the fifth the thorax, the sixth the front half of the abdomen, the seventh the rear half of the abdomen, and the eighth various other parts of the abdomen. It was not just that the first genes defined the head end of the fly and the last genes made the rear end of the fly. They were all laid out in order along the chromosome - without exception.
To appreciate how odd this was, you must know how random the order of genes usually is. In this book, I have told the story of the genome in a sort of logical order, picking genes to suit my purpose chapter by chapter. But I have deceived you a little in doing this: there is very little rhyme or reason for where a gene lies.
Sometimes it needs to be close to certain other genes. But it is surely rather literal of Mother Nature to lay these homeotic genes out in the order of their use.
A second surprise was in store. In 1983 a group of scientists working in Walter Gehring's laboratory in Basel discovered something common to all these homeotic genes. They all had the same 'paragraph' of text, 180 'letters' long, within the gene - known as the homeobox. At first, this seemed irrelevant. After all, if it was the same in every gene, it could not tell the fly to grow a leg rather than an antenna. All electrical appliances have plugs, but you cannot tell a toaster from a lamp by looking at the plug.
But the homeobox none the less enabled geneticists to go looking for other homeotic genes, like a tinker rooting through a pile of junk in search of anything with a plug attached. Gehring's colleague Eddie de Robertis, acting on no more than a hunch, went fishing among the genes of frogs for a 'paragraph' that looked like the homeobox. He found it. When he looked in mice, there it was again: almost exactly the same 180-letter string - the homeobox. Not only that, the mouse also turned out to have clusters of Hox genes (four of them, rather than one) and, in the same way as the fruit fly, the genes in the clusters were laid out end-to-end with the head genes first and the tail genes last.
What was doubly strange was that the mouse genes were recognisably the same genes as the fruit-fly genes.
There are differences, to be sure. Mice have thirty-nine Hox genes altogether, in four clusters, and they have up to five extra Hox genes at the rear end of each cluster that flies do not have. Various genes are missing in each cluster. But the similarity is still mind-blowing. It was so mind-blowing when it first came to light that few embryologists believed it. There was widespread scepticism, and belief that some silly coincidence had been exaggerated. One scientist remembers that on first hearing this news he dismissed it as another of Walter Gehring's wild ideas; it soon dawned on him that Gehring was being serious. John Maddox, editor of the journal Nature, called it 'the most important discovery this year (so far)'. At the level of embryology we are glorified flies. Human beings have exactly the same Hox clusters as mice, and one of them, Cluster C, is right here on chromosome 12.
we are descended from a common ancestor with flies which used the same way of defining the pattern of the embryo more than 530 million years ago, and that the mechanism was so good that all this dead creature's descendants have hung on to it.
Flies and people are just variations on a theme of how to build a body that was laid down in some worm-like creature in the Cambrian period. They still retain the same genes doing the same job. Of course, there are differences; if there were not, we would look like flies. But the differences are surprisingly subtle.
ndeed, so close are the similarities between genes that geneticists can now do, almost routinely, an experiment so incredible that it boggles the mind. They can knock out a gene in a fly by deliberately mutating it, replace it by genetic engineering with the equivalent gene from a human being and grow a normal fly. The technique is known as genetic rescue. Human Hox genes can rescue their fly equivalents, as can Otx and Emx genes. Indeed, they work so well that it is often impossible to tell which flies have been rescued with human genes and which with fly genes.
Even after 5 30 million years of separation, our computer can recognise a fly's software and vice versa.
From the basic asymmetry of chemicals injected into the egg all else follows. Genes turn each other on, giving the embryo a head and a rear. Other genes then get turned on in sequence from bow to stern giving each compartment an identity. Other genes then polarise the compartments into front and rear halves. Other genes then interpret all this information and make ever more complicated appendages and organs. It is a rather basic, chemical—mechanical, step-by-step process that would have appealed more to Aristotle than Socrates. From simple asymmetry can grow intricate pattern. Indeed, so simple is embryonic development in principle - though not in detail — that it is tempting to wonder if human engineers should not try to copy it, and invent self-assembling machines.
C H R O M O S O M E 13
P r e - H i s t o r y
Where invading men have imposed their language upon a land but married the local women, there should be a distinct set of Y-chromosome genes but a less distinct set of other genes. This is the case in Finland. The Finns are genetically no different from the other western Europeans who surround them, except in one notable respect: they have a distinct Y chromosome, which looks much more like the Y chromosome of northern Asian people.
Iceland is the perfect genetic laboratory because it was settled by such a small group of Norwegians around AD 900, and has seen so little immigration since. Virtually all of the 270,000 Icelanders trace their descent in all lines from those few thousand Vikings who reached Iceland before the little ice age. Eleven hundred years of chilly solitude and a devastating fourteenth-century plague have rendered the island so inbred that it is a happy genetic hunting ground.
A different mutation in the same gene, the deletion of the 6,174th 'letter', is common in people of Ashkenazi Jewish descent. Approximately eight per cent of Jewish breast-cancer cases under the age of forty-two are attributable to this one mutation.
Jewish people retained their genetic integrity by adding few converts to the faith and losing many people who married outsiders. As a result, the Ashkenazim in particular are a favourite people for genetic studies. In the United States the Committee for the Prevention of Jewish Genetic Disease organises the testing of schoolchildren's blood. When matchmakers are later considering a marriage between two young people, they can call a hotline and quote the two anonymous numbers they were each assigned at the testing. If they are both carriers of the same mutation, for Tay-Sachs disease or cystic fibrosis, the committee advises against the marriage. The practical results of this voluntary policy — which was criticised in 1993 by the New York Times as eugenic — are already impressive. Cystic fibrosis has been virtually eliminated from the Jewish population in the United States.
A similar story is taught by a gene on chromosome 1, the gene for lactase. This enzyme is necessary for the digestion of lactose, a sugar abundant in milk. We are all born with this gene switched on in our digestive system, but in most mammals - and therefore in most people — it switches off during infancy. This makes sense: milk is something you drink in infancy and it is a waste of energy making the enzyme after that.
Occasionally, however, the control gene which switches off the lactase gene must suffer a mutation and the lactase production fails to cease at the end of infancy. This mutation allows its carrier to drink and digest milk all through life. Fortunately for the makers of Corn Flakes and Weetabix, most western people have acquired the mutation. More than seventy per cent of western Europeans by descent can drink milk as adults, compared with less than thirty per cent of people from parts of Africa, eastern and south-eastern Asia and Oceania.
The Tutsi of central Africa, the Fulani of western Africa, the Bedouin, Tuareg and Beja of the desert, the Irish, Czech and Spanish people — this list of people has almost nothing in common except that all have a history of herding sheep, goats or cattle. They are the champion milk digesters of the human race. The evidence suggests that such people took up a pastoral way of life first, and developed milk-digesting ability later in response to it.
C H R O M O S O M E 1 4
I m m o r t a l i t y
There is an old story of a king who promised to reward a mathematician for some service with anything he wanted. The mathematician asked for a chessboard with one grain of rice on the first square, two on the second, four on the third, eight on the fourth and so on. By the sixty-fourth square, he would need nearly twenty million million million grains of rice, an impossibly vast number.
The story starts with a chance observation in 1972 by James Watson, DNA' s co-discoverer. Watson noticed that the biochemical machines that copy DNA, called polymerases, cannot start at the very tip of a DNA strand. They need to start several 'words' into the text. Therefore the text gets a little shorter every time it is duplicated. Imagine a photocopier that makes perfect copies of your text but always starts with the second line of each page and ends with the penultimate line. The way to cope with such a maddening machine would be to start and end each page with a line of repeated nonsense that you do not mind losing. This is exactly what chromosomes do. Each chromosome is just a giant, supercoiled, foot-long DNA molecule, so it can all be copied except the very tip of each end. And at the end of the chromosome there occurs a repeated stretch of meaningless 'text': the 'word' TTAGGG repeated again and again about two thousand times.
But every time the chromosome is copied, a little bit of the telomere is left off. After a few hundred copyings, the chromosome is getting so short at the end that meaningful genes are in danger of being left off. In your body the telomeres are shortening at the rate of about thirty-one 'letters' a year - more in some tissues. That is why cells grow old and cease to thrive beyond a certain age.
The reason that genes do not get left off in egg cells and sperm cells, the direct ancestors of the next generation, is the presence of telomerase, whose job is to repair the frayed ends of chromosomes, re-lengthening the telomeres.
In this context, note that the 'phrase' TTAGGG, which is repeated a few thousand times in each telomere, is exactly the same in the telomeres of all mammals. Indeed, it is the same in most animals, and even in protozoans, such as the trypanosome that causes sleeping sickness, and in fungi such as Neurospora. In plants the phrase has an extra T at the beginning: TTTAGGG. The similarity is too close to be coincidental. Telomerase has been around since the dawn of life, it seems, and has used almost the same RNA template in all descendants. Curiously, however, the ciliate protozoans — busy microscopic creatures covered in self-propelling fur - stand out as having a somewhat different phrase repeated in
their telomeres, usually TTTTGGGG or TTGGGG. The ciliates, you may remember, are the organisms that most frequently diverge from the otherwise-universal genetic code. More and more evidence points to the conclusion that the ciliates are peculiar creatures that
do not fit easily into the files of life.
Telomerase seems to behave like the elixir of eternal life for cells. Geron Corporation, a company devoted to telomerase research, was founded by the scientist who first showed that telomeres shrink in dividing cells, Cal Harley. Geron hit the headlines in August 1997 for cloning part of telomerase. Its share price promptly doubled, not so much on the hope that it could give us eternal youth as on the prospect of making anti-cancer drugs: tumours require telomerase to keep them growing. But Geron went on to immortalise cells with telomerase. In one experiment, Geron scientists took two cell types grown in the laboratory, both of which lacked natural telomerase, and equipped them with a gene for telomerase. The cells continued dividing, vigorous and youthful, far beyond the point where they would normally senesce and die. At the time the result was published the cells that had had the telomerase gene introduced had exceeded their expected lifespan by more than twenty doublings, and they showed no sign of slowing down.
Every animal has roughly the same number of heartbeats per lifetime. An elephant lives longer than a mouse, but its pulse rate is so much slower that, measured in heartbeats, they both live lives of the same length.
There is a famous pair of photographs of the Scottish ornithologist George Dunnet holding the same wild fulmar petrel in 1950 and 1992. The fulmar looks exactly the same in the two pictures; Professor Dunnet doesn't.
The reason it lives far longer than a mouse is because in the life of the fulmar there is no equivalent of the cat and the owl: no natural predators. A mouse is unlikely to make it past three years of age, so genes that damage four-year-old mouse bodies are under virtually no selection to die out.
Evidence for this theory comes from a natural experiment studied by Steven Austad on an island called Sapelo, which lies about five miles off the coast of Georgia in the United States. Sapelo contains a population of Virginia opossums that has been isolated for 10,000 years. Opossums, like many marsupials, age very rapidly. By the age of two years, opossums are generally dead from old age - the victims of cataracts, arthritis, bare skin and parasites. But that hardly matters because by two they have generally been hit by a truck, a coyote, an owl or some other natural enemy. On Sapelo, reasoned Austad, where many predators are absent, they would live longer and so — exposed for the first time to selection for better health after two years of age - their bodies would deteriorate less rapidly. They would age more slowly. This proved an accurate prediction. On Sapelo, Austad found, the opossums not only lived much longer, but aged more slowly. They were healthy enough to breed successfully in their second year - rare on the mainland — and their tendons showed less stiffness than those in mainland opossums. The evolutionary theory of ageing explains all the cross-species trends in a satisfying way. It explains why slow-ageing species tend to be large (elephants), or well protected (tortoises, porcupines), or relatively free from natural predators (bats, seabirds). In each case, because the death rate from accidents or predation is low, so the selective pressure is high for versions of genes that prolong health into later life.
All our systems begin to break down at once, as in the old story of the Detroit car maker who employed somebody to go around breakers' yards finding out which parts of cars did not break down, so that those bits could in future be made to a lower specification. Natural selection has designed all parts of our bodies to last just long enough to see our children into independence, no more.
It is no accident that the immortal cell lines used by scientists in the laboratory are derived from cancer patients. The most famous of them, the HeLa cell line, originated in the cervical tumour of a patient named Henrietta Lacks, a black woman who died in Baltimore in 1951. Her cancer cells are so wildly proliferative when cultured in the laboratory that they often invade other laboratory samples and take over the Petri dish.
HeLa cells were used for developing polio vaccines and have gone into space. Worldwide, they now weigh more than 400 times Henrietta's own body weight. They are spectacularly immortal.
C H R O M O S O M E 1 5
S e x
Harry Angelman was a doctor working in Warrington in Lancashire when he first realised that rare cases of what he called 'puppet children' were suffering from an inherited disease. In contrast to those with Prader-Willi syndrome, they are not floppy, but taut. They are thin, hyperactive, insomniac, small-headed and long-jawed, and often stick out their large tongues. They move jerkily, like puppets, but have a happy disposition; they are perpetually smiling and are given to frequent paroxysms of laughter. But they never learn to speak and are severely mentally retarded. Angelman children are much rarer than Prader-Willi children, but they sometimes crop up in the same family tree. In both Prader-Willi and Angelman's syndrome it soon became clear that the same chunk of chromosome 15 was missing. The difference was that in Prader-Willi syndrome, the missing chunk was from the father's chromosome, whereas in Angelman's syndrome, the missing chunk was from the mother's chromosome. Transmitted through a man, the disease manifests itself as Prader-Willi syndrome; transmitted through a woman it manifests itself as Angelman's syndrome. These facts fly in the face of everything we have learnt about genes since Gregor Mendel. They seem to belie the digital nature of the genome and imply that a gene is not just a gene but carries with it some secret history of its origin. The gene 'remembers' which parent it came from because it is endowed at conception with a paternal or a maternal imprint — as if the gene from one parent were written in italic script. In every cell where the gene is active, the 'imprinted' version of the gene is switched on and the other version switched off.
In the late 1980s, two groups of scientists, one in Philadelphia and one in Cambridge, made a surprising discovery. They tried to create a uniparental mouse — a mouse with only one parent. Since strict cloning from a body cell was then impossible in mice (post- Dolly, this is quickly changing), the Philadelphia team swapped the 'pronuclei' of two fertilised eggs. When an egg has been fertilised by a sperm, the sperm nucleus containing the chromosomes enters the egg but does not at first fuse with the egg nucleus: the two nuclei are known as 'pronuclei'. A clever scientist can sneak in with his pipette and suck out the sperm pronucleus, replacing it with the egg pronucleus from another egg — and vice versa. The result is two viable eggs, but one with, genetically speaking, two fathers and no mother and the other with two mothers and no father. The Cambridge team used a slightly different technique to reach the same result. But in both cases such embryos failed to develop properly and soon died in the womb. In the two-mothers case, the embryo itself was properly organised, but it could not make a placenta with which to sustain itself. In the two-fathers case, the embryo grew a large and healthy placenta and most of the membranes that surround the foetus. But inside, where the embryo should be, there was a disorganised blob of cells with no discernible head.
These results led to an extraordinary conclusion. Paternal genes, inherited from the father, are responsible for making the placenta; maternal genes, inherited from the mother, are responsible for making the greater part of the embryo, especially its head and brain. Why should this be? Five years later, David Haig, then at Oxford, thought he knew the answer. He had begun to reinterpret the mammalian placenta, not as a maternal organ designed to give sustenance to the foetus, but more as a foetal organ designed to parasitise the maternal blood supply and brook no opposition in the process. He noted that the placenta literally bores its way into the mother's vessels, forcing them to dilate, and then proceeds to produce hormones which raise the mother's blood pressure and blood sugar. The mother responds by raising her insulin levels to combat this invasion. Yet, if for some reason the foetal hormone is missing, the mother does not need to raise her insulin levels and a normal pregnancy ensues. In other words, although mother and foetus have a common purpose, they argue fiercely about the details of how much of the mother's resources the foetus may have — exactly as they later will during weaning.
Imprinting is a feature of placental mammals and of plants whose seeds gain sustenance from the parent plant.
If imprinted genes exist only to combat each other, then you should be able to switch both off and it will have no effect at all on the development of the embryo. You can. Elimination of all imprinting leads to normal mice.
Imprinting has a curious consequence. In a man, the maternal copy of chromosome 15 carries a mark that identifies it as coming from his mother, but when he passes it on to his son or daughter, it must somehow have acquired a mark that identifies it as coming from him: the father. It must switch from maternal to paternal and vice versa in the mother. That this switch does happen we know, because in a small proportion of people with Angelman syndrome there is nothing unusual about either chromosome except that both behave as if they were paternal. These are cases in which the switch failed to occur.
The mark consists of one gene's methylation. But methylation is removed during the early development of the embryo - the creation of the so-called blastocyst — and then reimposed during the next stage of development, called gastrulation. Somehow, imprinted genes escape this process. They resist the demethylation.
That imprinted genes escape demethylation is, we now know, all that stood between science and the cloning of mammals for many years. Toads were fairly easily cloned by putting genes from a body cell into a fertilised egg, but it just didn't work with mammals, because the genome of a female's body cells had certain critical genes switched off by methylation and the genome of a male's body cells had other genes switched off - the imprinted genes. So, confidently, scientists followed the discovery of imprinting with the announcement that cloning a mammal was impossible. A cloned mammal would be born with all its imprinted genes either on or off on both chromosomes, upsetting the doses required by the cells of the animal and causing development to fail. 'A logical consequence', wrote the scientists who discovered imprinting,10 'is the unlikelihood of successful cloning of mammals using somatic cell nuclei.'
Then, suddenly, along came Dolly the cloned Scottish sheep in early 1997. Quite how she and those that came after evaded the imprinting problem remains a mystery, even to her creators, but it seems that a certain part of the treatment meted out to her cells during the procedure erased all genetic imprints.
When an egg is formed inside a woman's ovary, it usually receives one copy of each chromosome, but in rare cases where a pair of parental chromosomes fails to separate, the egg ends up with two copies. After fertilisation with a sperm, the embryo now has three copies of that chromosome, two from the mother and one from the father. This is especially likely in elder mothers, and is generally fatal to the egg. The embryo can go on to develop into a viable foetus and survive more than a few days after birth only if the triplicate chromosome is number 21, the smallest of the chromosomes - the result being Down syndrome. In other cases the extra chromosome would so upset the biochemistry of the cells that development would fail. However, in most cases, before that stage is reached, the body has a way of dealing with this triplet problem. It 'deletes' one chromosome altogether, leaving two, as intended. The difficulty is that it does so at random. It cannot be sure that it is deleting one of the two maternal chromosomes, or the single paternal one. Random deletion has a sixty-six per cent chance of getting one of the maternal ones, but accidents do happen. If, by mistake, it deletes the paternal one, then the embryo goes merrily on its way with two maternal chromosomes. In most cases this could not matter less, but if the tripled chromosome is number 15, you can see immediately what will ensue. Two copies of UBE3A, the maternally imprinted gene, are expressed, and no copies of SNRPN, the paternally imprinted gene. The result is Prader-Willi syndrome.
Chimeras are fused bodies of two genetically distinct individuals. They occur naturally — you may have met some or even be one yourself, though you will not know it without a detailed study of the chromosomes. Two genetically distinct embryos happen to fuse together and grow as if they were one. Think of them as the opposite of identical twins: two different genomes in one body, instead of two different bodies with the same genome. It is comparatively easy to make mouse chimeras in the laboratory by gently fusing the cells from two early embryos. But what the ingenious Cambridge team did in this case was to fuse a normal mouse embryo with an embryo that was made by 'fertilising' an egg with another egg's nucleus, so that it had purely maternal genes and no contribution from the father. The result was a mouse with an unusually large head. When these scientists made a chimera between a normal embryo and an embryo derived only from the father (i.e., grown from an egg whose nucleus had been replaced by two sperm nuclei), the result was the opposite: a mouse with a big body and a small head.
Most of the striatum, cortex and hippocampus of the mouse brain are consistently made by these maternal cells, but that such cells are excluded from the hypothalamus. The cortex is the place where sensory information is processed and behaviour is produced. Paternal cells, by contrast, are comparatively scarce in the brain, but much commoner in the muscles. Where they do appear in the brain, however, they contribute to the development of the hypothalamus, amygdala and preoptic area. These areas comprise part of the 'limbic system' and are responsible for the control of emotions.
In the 1960s, in the United States, a botched circumcision left a boy with a badly damaged penis, which the doctor decided to amputate. It was decided to try to turn the boy into a girl by castration, surgery and hormonal treatment. John became Joan; she wore dresses and played with dolls. She grew up into a young woman. In 1973 John Money, a Freudian psychologist, claimed in a burst of publicity that Joan was a well adjusted adolescent, and her case thus put an end to all speculation: gender roles were socially constructed. Not until 1997 did anybody check the facts. When Milton Diamond and Keith Sigmundson tracked down Joan, they found a man, happily married to a woman. His story was very different from that told by Money. He had always felt deeply unhappy about something as a child and had always wanted to wear trousers, mix with boys and urinate standing up. At the age of fourteen he was told by his parents what had happened, which brought great relief. He ceased hormonal treatment, changed his name back to John, resumed the life of a man, had his breasts removed and at the age of twenty-five married a woman and adopted her children. Held up as a proof of socially constructed gender roles, he proved the exact opposite: that nature does play a role in gender.
C H R O M O S O M E 16
M e m o r y
Dunce is a mutant fruit fly incapable of learning that a certain smell is always followed by an electric shock. Discovered in the 1970s, it was the first of a string of 'learning mutants' to be discovered by giving irradiated flies simple tasks to learn and breeding from those that could not manage the tasks.
By removing the gene for the CREB protein, he created a fly that could learn, but not remember that it had learnt - the lesson soon faded from its memory.
By systematically searching for other mutant flies incapable of learning or memory, Ronald Davis, Michael Grotewiel and their colleagues in Houston came up with a different kind of mutant fly, which they called volado. ('Volado', they helpfully explain, is a Chilean colloquialism meaning something akin to 'absent-minded' or 'forgetful', and generally applied to professors.)
They took some flies in which the volado gene had been knocked out, and inserted a fresh copy linked with a 'heat-shock' gene - a gene that becomes switched on when suddenly heated up. They had carefully arranged the two so that the volado gene only worked when the heat-shock gene was on. At cool temperatures, the flies could not learn. Three hours after a heat shock, however, they suddenly became good learners. A few hours after that, as the heat shock faded into the past, they again lost the ability to learn. This means that volado is needed at the exact moment of learning; it is not just a gene required to build the structures that do the learning. The fact that the volado gene's job is to make a protein that binds cells together raises the intriguing hint that memory may consist, quite literally, of the tightening of the connections between neurons.
When you learn something, you alter the physical network of your brain so as to create new, tight connections where there were none or weaker ones before.
A pyramidal neuron is difficult to 'fire', but if two separate inputs arrive at once, their combined effect will fire it. Once fired, it is much easier to fire but only by one of the two inputs that originally fired it, and not by another input. Thus, the sight of a pyramid and the sound of the word 'Egypt' can combine to fire a pyramidal cell, creating an associative memory between the two, but the thought of a sea-horse, although perhaps connected to the same pyramidal cell, is not 'potentiated' in the same way because it did not coincide in time.
That is an example of long-term potentiation. If you think, too simplistically, of the pyramidal cell as the memory of Egypt, then it can now be fired by the word or the picture, but not by a sea-horse.
C H R O M O S O M E 1 7
D e a t h
Prodded by genes like ced-9, the unneeded cells commit mass suicide (other ced genes cause suicide in other body tissues). The dying cells obediently follow a precise protocol. In microscopic nematode worms, the growing embryo eventually contains 1,090 cells, but precisely 131 of these kill themselves during development, leaving 959 cells in an adult worm. It is as if they sacrifice themselves for the greater good of the body. 'Dulce et decorum est pro corpore mori'' they cry and fade heroically away, like soldiers going over the top at Verdun, or worker bees suicidally stinging an intruder. The analogy is far from specious. The relationship between body cells is indeed very much like that between bees in a hive. The ancestors of your cells were once individual entities and their evolutionary 'decision' to co-operate, some 600 million years ago, is almost exactly equivalent to the same decision, taken perhaps fifty million years ago by the social insects, to co-operate on the level of the body: close genetic relatives discovered they could reproduce more effectively if they did so vicariously, delegating the task to germ cells in the cells' case, or to a queen in the case of bees. The analogy is so good that evolutionary biologists have begun to realise that the co-operative spirit goes only so far. Just as soldiers at Verdun were occasionally driven to mutiny against the greater good, so worker bees are capable of reproducing on their own if they get the chance; only the vigilance of other workers prevents them. The queen buys the loyalty of those other workers to herself rather than to their sister workers by mating with several males to ensure that most workers are only half-sisters of each other and therefore share little genetic common interest. And so it is with cells in the body. Mutiny is a perpetual problem. Cells are continually forgetting their patriotic duty, which is to serve the germ cells, and
setting out to reproduce themselves. After all, each cell is descended from a long line of reproducing cells; it goes against the grain to cease dividing for a whole generation. And so, in every tissue every day, there is a cell that breaks ranks and starts to divide again, as if unable to resist the age-old call of the genes to reproduce themselves. If the cell cannot be stopped, we call the result cancer. But usually, it can be stopped. The problem of cancerous mutiny is so old that in all large bodied animals the cells are equipped with an elaborate series of switches designed to induce the cell to commit suicide if it should find itself turning cancerous. The most famous and important of these switches, in fact possibly the most talked about of all human genes since its discovery in 1979, is TP53, which lies on the short arm of chromosome 17. This chapter tells the remarkable story of cancer, through the eyes of a gene whose principal job is to prevent it.
The longer we live, the more mistakes we accumulate in our genes, and the greater the chance that an oncogene may be jammed on and three tumour-suppressor genes jammed off in the same cell.
Mainly by switching on other genes, p53 tells the cell to do one of two things: either to halt proliferation, stop replicating its DNA and pause until repaired; or to kill itself.
TP53 seems to encode the greater good, like a suicide pill in the mouth of a soldier that dissolves only when it detects evidence that he is about to mutiny. The suicide of cells in this way is known as apoptosis, from the Greek for the fall of autumn leaves. It is the most important of the body's weapons against cancer, the last line of defence.
These treatments do indeed cause a little DNA damage, he says, but not enough to kill the cells. Instead, the DNA damage is just sufficient to alert p53, which tells the cells to commit suicide. So chemotherapy and radiation therapy are actually, like vaccination, treatments that work by helping the body to help itself.
If a cell detects that it has been infected with a virus, it can kill itself for the good of the body as a whole (ants and bees may do this as well, for the good of their colonies). There is good evidence that some cells do indeed do exactly this. There is also, inevitably, evidence that some viruses have evolved a way of preventing this from happening. Epstein¬ Barr virus, the cause of glandular fever or mononucleosis, contains a latent membrane protein whose job seems to be to head off any tendency the infected cell shows to commit suicide. Human papilloma virus, cause of cervical cancer, has two genes aboard whose job is to switch off TP53 and another tumour-suppressor gene.
Apoptosis is a decentralised business. There is no central planning, no bodily Politburo deciding who should die and who should live. That is the beauty of it. Like the development of the embryo, it harnesses the self-knowledge of each cell. There is only one conceptual difficulty: how apoptosis could have evolved. In passing the test of killing itself if infected, cancerous or genetically mischievous, a cell by definition dies. It cannot therefore pass on its goodness to its daughters. Known as 'the kamikaze conundrum', this problem is solved by a form of group selection: whole bodies in which apoptosis works well do better than whole bodies in which it fails to work; the former therefore pass on the right traits to the cells of their offspring. But it does mean that the apoptotic system cannot improve during a person's lifetime, because it cannot evolve by
natural selection within the body. We are stuck with the cell-suicide mechanism that we inherited.
C H R O M O S O M E 18
C u r e s
'What's the rush?' asked Anderson at one session. 'A patient dies of cancer every minute in this country. Since we began this discussion 146 minutes ago, 146 patients have died of cancer.'
At their birth, new technologies often seem hopelessly uncompetitive. The first railways were far more expensive than the existing canals and far less reliable. Only gradually and with time does the new invention bring down its own costs or raise its efficacy to the point where it can match the old.
In 1992 Kenneth Culver tried an audacious experiment that involved the first direct injection of gene-equipped retroviruses into the human body (as opposed to infection of cultured cells outside the body and transfusion of those cells back in). He injected retroviruses directly into brain tumours of twenty people. Injecting anything into the brain sounds horrifying enough, let alone a retrovirus. But wait till you hear what was in the retrovirus. Each one was equipped with a gene taken from a herpes virus. The tumour cells took up the retrovirus and expressed the herpes gene. But by then the cunning Dr Culver was treating the patient with drugs for herpes; the drugs attacked the tumours. It seemed to work on the first patient, but on four of the next five it failed.
Transgenic mice are scientific gold dust. They enable scientists to find out what genes are for and why. The inserted gene need not be derived from a mouse, but could be from a person: unlike in computers, virtually all biological bodies can run any kind of software.
Sheep have already been given the gene for a human clotting factor in the hope that it can be harvested from their milk and used to treat haemophiliacs. (Almost incidentally, the scientists who performed this procedure cloned the sheep Dolly and displayed her to an amazed world in early 1997.) A company in Quebec has taken the gene that enables spiders to make silk webs and inserted it into goats, hoping to extract raw silk protein from the goats' milk and spin it into silk.
C H R O M O S O M E 1 9
P r e v e n t i o n
You may be wondering why E4 exists at all, let alone at such high frequencies. If it exacerbates both heart disease and Alzheimer's, it should surely have been driven extinct by the more benign E3 and E2 long ago. I'm tempted to answer the question by saying that high-fat diets were until recently so rare that the coronary side-effects were of little importance, while Alzheimer's disease is all but irrelevant to natural selection, since it not only happens to people who have long ago reared their own children to independence, but strikes at an age when most Stone-Age folk were long dead anyway.
The difference between E4 and the commoner E3 is that the 334th 'letter' in the gene is G instead of A. The difference between E3 and E2 is that the 472nd 'letter' is a G instead of an A. The effect is to give E2's protein two extra cysteines and E4's two extra arginines compared with each other, E3 being intermediate. These tiny changes in a gene that is 897 'letters' long are sufficient to alter the way APOE's protein does its job.
If genetic testing were to become routine for lots of genes, the entire concept of pooled risk, on which insurance is based, would be undermined. Once my exact fate is known, I would be quoted a premium that covered the exact cost of my life.
Politics
The story starts with sheep. In eighteenth-century Britain, agricul- ture was revolutionised by a group of pioneering entrepreneurs, among them Robert Bakewell of Leicestershire. It was Bakewell's discovery that sheep and cattle could be rapidly improved by select- ively breeding the best specimens with their own offspring to concentrate desirable features. Applied to sheep this inbreeding pro- duced fast-growing, fat lambs with long wool. But it had an unexpec- ted side-effect. Sheep of the Suffolk breed, in particular, began to exhibit symptoms of lunacy in later life. They scratched, stumbled, trotted with a peculiar gait, became anxious and seemed antisocial. They soon died. This incurable disease, called scrapie, became a large problem, often killing one ewe in ten. The scrapie followed Suffolk sheep, and to a lesser extent other breeds, to other parts of the world. Its cause remained mysterious. The disease did not seem to be inherited, but it could not be traced to another origin.
About this time an American scientist, Bill Hadlow saw pictures of the damaged brains of scrapie-riddled sheep in an exhibit in the Wellcome Museum of Medicine in London. He was struck by their similarity to pictures he had seen from a very different place. Scrapie was about to get a lot more relevant to people. The place was Papua New Guinea, where a terrible debilitating disease of the brain, known as kuru, had been striking down large numbers of people, especially women, in one tribe known as the Fore. First, their legs began to wobble, then their whole bodies started to shake, their speech became slurred and they burst into unexpected laughter. Within a year, as the brain progressively dissolved from within, the victim would be dead. By the late 1950s, kuru was the leading cause of death among Fore women, and it had killed so many that men outnumbered women by three to one. Children also caught the disease, but comparatively few adult men.
This proved a crucial clue. In 1957 Vincent Zigas and Carleton Gajdusek, two western doctors working in the area, soon realised what had been happening. When somebody died, the body was ceremonially dismembered by the women of the tribe as part of the funeral ritual and, according to anecdote, eaten. Funereal cannibalism was well on the way to being stamped out by the government, and it had acquired sufficient stigma that few people were prepared to talk openly about it. This has led some to question whether it ever happened. But Gajdusek and others gathered sufficient eye-witness accounts to leave little doubt that the Fore were not lying they described pre-1960 funeral rituals in Pidgin as 'katim na kukim na kaikai' - or cut up, cook and eat. Generally, the women and children ate the organs and brains; the men ate the muscle. This immediately suggested an explanation for kuru's pattern of appear- ance. It was commonest among women and children; it appeared among relatives of victims - but among married relations as well as blood relatives; and after cannibalism became illegal, the age of its victims steadily increased. In particular, Robert Klitzman, a student of Gajdusek's, identified three clusters of deaths, each of which included only those who attended certain funerals of kuru victims in the 1940s and 1950s. For instance, at the funeral of a woman called Neno in 1954, twelve of fifteen relatives who attended later died of kuru. The three who did not comprised somebody who died young of another cause, somebody who was forbidden by tradition to take part in the eating because she was married to the same man as the dead woman, and somebody who later claimed to have eaten only a hand.
PRP is a normal gene in mice and people; it produces a normal protein. It is not the gene of a virus. But its product, known as a prion, is a protein with an unusual quality: it can suddenly change its shape into a tough and sticky form that resists all attempts to destroy it and that gathers together in aggregate lumps, disrupting the structure of the cell. All this would be unprecedented enough, but Prusiner proposed something even more exotic. He suggested that this new form of prion has the capacity to reshape normal prions into versions of itself. It does not alter the sequence — proteins, like genes, are made of long, digital sequences - but it does change the way they fold up.
The PRP gene is not only present in every mammal so far examined, but it varies very little in sequence, which implies that it is doing some important job. That job almost certainly concerns the brain, which is where the gene is switched on. It may involve copper, which the prion seems to be fond of. But - and here's the mystery — a mouse in which both copies of the gene have been deliberately knocked out since before birth is a perfectly normal mouse. It seems that whatever function the prion serves, the mouse can grow to do without it. We are still no nearer to knowing why we have this potentially lethal gene.
If anything, CJD is even more precise than Huntington's disease in the age at which it strikes. The record includes cases of siblings who caught it at exactly the same age despite living apart all their lives.
CHROMOSOME 21
Eugenics
Children are occasionally born with an extra chromosome 13 or 18, but they never survive more than a few days. Children born with an extra chromosome 21 are healthy, conspicuously happy and destined to live for many years. But they are not considered, in that pejorative word, 'normal'. They have Down syndrome.
At a time of rapid immigration from eastern and southern Europe, it was easy to whip up paranoia that the 'better' Anglo-Saxon stock of the country was being diluted. Eugenic arguments provided a convenient cover for those who wished to restrict immigration for more traditional, racist reasons. The Immigration Restriction Act of 1924 was a direct result of eugenic campaigning.
In Buck v. Bell, the court ruled that the commonwealth of Virginia could sterilise Carrie Buck, a seventeen- year-old girl committed to a colony for epileptics and the feeble minded in Lynchburg, where she lived with her mother Emma and her daughter Vivian. After a cursory examination, Vivian, who was seven months old (!), was declared an imbecile and Carrie was ordered to be sterilised. As Justice Oliver Wendell Holmes famously put it in his judgment, 'Three generations of imbeciles are enough.' Vivian died young, but Carrie survived into old age, a respectable woman of moderate intelligence who did crossword puzzles in her spare time. Her sister Doris, also sterilised, tried for many years to have babies before realising what had been done to her without her consent.
The clause that really stuck in Wedgwood's throat was the one that stated it to be 'desirable in the interests of the community that [the feeble-minded] should be deprived of the opportunity of procreating children.' This was, in Wedgwood's words, 'the most abominable thing ever suggested' and not 'the care for the liberty of the subject and for the protection of the individual against the state that we have a right to expect from a Liberal Administration'.
Wedgwood probably thought he had failed. The forcible commit- tal of mental patients became a feature of British life and this in practice did make it harder for them to breed. But in truth he had not only prevented eugenic measures being adopted; he had also sent a warning shot across the bows of any future government that eugenic legislation could be contentious. And he had identified the central flaw in the whole eugenic project. This was not that it was based on faulty science, nor that it was impractical, but that it was fundamentally oppressive and cruel, because it required the full power of the state to be asserted over the rights of the individual.
What is wrong with eugenics is not the science, but the coercion.
CHROMOSOME 22
Free Will
It used to be conventional wisdom that distant fathers and over-protective mothers turn sons gay. It is now considered much more likely to be the reverse: perceiving that a son is not fully interested in masculine concerns, the father retreats; the mother compensates by being overprotective.
Stephen Mobley was convicted of the murder of a pizza-shop man- ager, John Collins, and sentenced to death. Appealing to have the sentence reduced to life imprisonment, his lawyers offered a genetic defence. Mobley came, they said, from a long pedigree of crooks and criminals. He probably killed Collins because his genes made him do it. 'He' was not responsible; he was a genetically determined automaton.